Large-scale computer simulation reveals how magnetic field lines break to produce solar flares and other phenomena
Suzette Chan - 25 March 2021
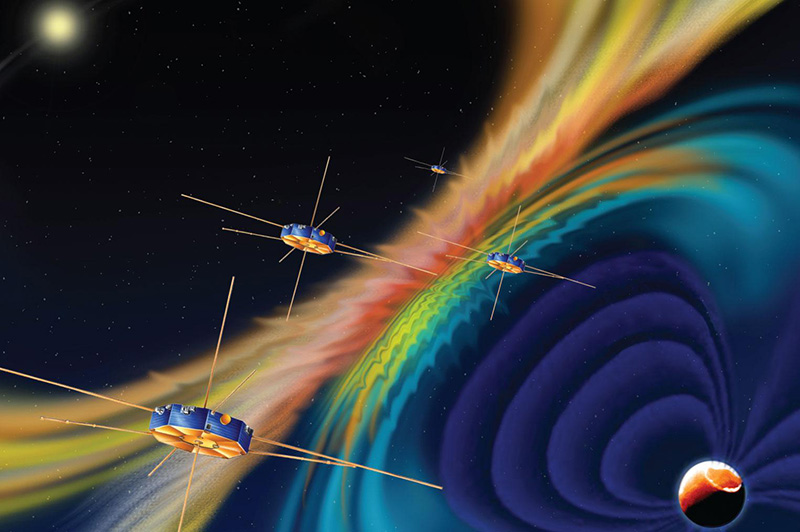
This artist rendition of the Magnetospheric Multiscale Mission (MMS) spacecraft shows the magnetic topology in the background. The solar magnetic field lines (orange-reddish colour) makes contact with the Earth's magnetic field (bluish colour). Where they meet, there is a 'magnetic explosion.' Waves and energized plasma then emanate from the magnetic reconnection zone. Image: NASA.
Solar flares, geomagnetic substorms (of which the aurora borealis is a manifestation), and gamma-ray bursts share a common cause: they happen when magnetic field lines in space break and reconnect. A University of Alberta astrophysicist has been studying the mechanics of this process and has just co-authored a paper with surprising findings.
“It is important to understand how magnetic field lines break and reconnect, since this process can rearrange the entire magnetic structure or topology surrounding a planetary body,” says professor Richard Sydora.
For instance, when magnetic field lines break just above the Sun’s surface and into the corona, large amounts of magnetic energy are released. The resulting solar flares eject energetic charged particles into space and toward the Earth. “This poses a serious danger to orbiting satellites and telecommunications,” says Sydora.
To help visualize magnetic fields, astrophysicists use vectors to depict a magnetic field line. Sydora says, “Since charged particles follow the magnetic field lines, like beads on a string, it also gives us the pattern of movement of the charged particles in the plasma.”
Sydora has been studying these dynamics of field lines for years. His frequent collaborator, professor Keizo Fujimoto at Beihang University, China, was a postdoctoral fellow under his supervision at the University of Alberta in 2006 to 2007. In 2012, the Physics Review Letter published their paper about the largest simulation on the magnetic energy release problem to that date.
Their work took a leap forward in 2014 to 2018, when they had access to the RIKEN Advanced Institute for Computational Science’s K computer, which was among the most powerful computers in the world at that time. They created a new technique for the numerical model, and applied it to a set of very large calculations in 2018.
“The largest run took around one month to complete,” Sydora recalls. “The data sets from these types of calculations are enormous! There was so much data to ‘swim’ through and process that it took a couple years to analyze and make sense.”
When they emerged, they found that the new numerical modelling technique and the increased computing capacity had led to a surprising discovery.
“The viscosity effects due to wave turbulence became evident and changed our view on how magnetic field lines break and reconnect, releasing all this energy in the process; previously electrical resistivity from wave turbulence was believed to dominate, however, this appears not to be the case,” Sydora says.
The paper gives some predictions of the types of “signatures” (waves and turbulence) researchers can look for in space plasma data to further investigate the viscosity effects. Currently, Sydora and Fujimoto are working on how to apply this viscosity in magnetohydrodynamics (MHD) models in order to test how the results of magnetic energy release could be modified.
Additional proof could be provided by NASA’s current Magnetospheric Multiscale Mission (MMS), which has four satellites, flying in tight formation around the Earth, with instruments that measure electric and magnetic fields along with local plasma energy and density, with the aim of uncovering the microphysics of magnetic reconnection. “Analysis of the data from this particular mission could lead to the near-term confirmation of our findings.”
Fujimoto and Sydora’s paper, “Electromagnetic turbulence in the electron current layer to drive magnetic reconnection”, was published in Astrophysical Journal Letters (doi: 10.3847/2041-8213/abe877).