Richard A. Rachubinski
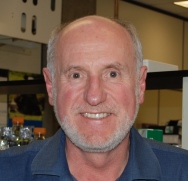
Richard A. Rachubinski
Ph.D., McGill University
Professor
Office: 6-50A Medical Sciences BuildingLaboratory: 6-50 Medical Sciences Building
Telephone: 780-492-9868
rick.rachubinski@ualberta.ca
Awards
- Distinguished University Professor
- Howard Hughes Medical Institute International Research Scholar
- Canada Research Chair in Cell Biology
- Canadian Institutes of Health Research Senior Investigator
- Fellow of the Royal Society of Canada
- Fellow of the Canadian Academy of Health Sciences
- Fellow of the American Association for the Advancement of Science
Molecular Mechanisms of Peroxisome Biogenesis
Peroxisomes are ubiquitous organelles that are defined as containing at least one hydrogen-peroxide-producing oxidase and catalase to decompose the hydrogen peroxide. They perform many biochemical functions, including the oxidation of fatty acids and the detoxification of reactive oxygen species. Mature peroxisomes are spherical, with diameters between 0.5 and 1.0 micrometer. Each peroxisome is delimited by a single membrane and contains a fine granular matrix. Peroxisomes do not contain DNA or an independent protein synthesis machinery. Accordingly, all peroxisomal proteins are encoded in the nucleus and synthesized on cytosolic polysomes.
Peroxisomes are essential for normal human development and physiology, as shown by the lethality of a group of genetic disorders called the peroxisome biogenesis disorders (PBDs). PBD-afflicted individuals fail to assemble functional peroxisomes. We are therefore interested in defining the molecular cascade of events involved in peroxisome biogenesis.
![]() |
Electron micrograph of a cell of the yeast |
We use the yeasts Yarrowia lipolytica and Saccharomyces cerevisiae and the fruit fly Drosophila to study peroxisome biogenesis and to model the PBDs.
Yeasts are good models for studying the molecular bases of the PBDs for two reasons. First, the mechanism of peroxisome assembly has been conserved during evolution from yeasts to humans. Second, there is a conditional requirement for peroxisomes on different carbon sources that makes yeasts readily tractable genetic model systems with which to study peroxisome biogenesis and has permitted the generation and identification of a number of peroxisome assembly, or pex, mutants that mimic the human PBDs. Complementation of these yeast pex mutants has played a paramount role in the identification of a large number of PEX genes encoding peroxins required for peroxisome assembly. These yeast genes have been used to identify human PEX gene orthologs. The PEX genes complementing fourteen complementation groups of the PBDs have now been identified.
Our current research in yeast combines global biological approaches like differential gene expression analysis and proteomics with bioinformatics and cell biological techniques like fluorescence microscopy, video microscopy and subcellular fractionation to identify novel proteins and mechanisms involved in peroxisome biogenesis, peroxisome population control, and peroxisome inheritance.
![]() |
Wild-type and PBD embryos of Drosophila
|
Yeast is, of course, under most conditions unicellular. We therefore developed the fruit fly Drosophila as a valid and tractable multi-cellular, multi-tissue model organism for the PBDs in collaboration with Dr. Andrew Simmonds in the Department of Cell Biology. We are using fruit fly to expand our knowledge of PBD pathophysiology, and to identify therapeutics and evaluate their efficacy in reducing the severity of symptoms of the PBDs.
Selected Publications
Banerjee, H. and Rachubinski, R.A. Involvement of SNARE protein Ykt6 in glycosome biogenesis in Trypanosoma brucei. Mol. Biochem. Parasitol. 218:28-37 (2017).
Di Cara, F., Sheshachalam, A., Braverman, N.E., Rachubinski, R.A. and Simmonds, A.J. Peroxisome-mediated metabolism is required for immune response to microbial infection. Immunity 47:93-106 (2017).
Mast, F.D., Jamakhandi, A., Saleem, R.A., Dilworth, D.J., Rogers, R.S., Rachubinski, R.A. and Aitchison, J.D. Peroxins Pex30 and Pex29 dynamically associate with reticulons to regulate peroxisome biogenesis from the endoplasmic reticulum. J. Biol. Chem. 291:15408-15427 (2016).
Knoblach, B. and Rachubinski, R.A. How peroxisomes partition between cells. A story of yeast, mammals and filamentous fungi. Curr. Opin. Cell Biol. 41:73-80 (2016).
Baron, M.N., Klinger, C.M., Rachubinski, R.A. and Simmonds, A.J. A systematic cell-based analysis of predicted Drosophila peroxisomal proteins. Traffic 17:536-553 (2016).
Chang, J., Klute, M.J., Tower, R.J., Mast, F.D., Dacks, J.B. and Rachubinski, R.A. An ancestral role in de novo peroxisome assembly is retained by the divisional peroxin Pex11 in the yeast Yarrowia lipolytica. J. Cell Sci. 128:1327-1340 (2015).
Knoblach, B. and Rachubinski, R.A. Transport and retention mechanisms govern lipid droplet inheritance in Saccharomyces cerevisiae. Traffic 16:298-309 (2015).
Chang, J., Tower, R.J., Lancaster, D.L. and Rachubinski, R.A. Dynein light chain interaction with the peroxisomal import docking complex modulates peroxisome biogenesis in yeast. J. Cell Sci. 126:4698-4706 (2013).
Knoblach, B., Sun, X., Coquelle, N., Fagarasanu, A., Poirier, R.L., and Rachubinski, R.A. An ER-peroxisome tether exerts peroxisome population control in yeast. EMBO J. 32:2439-2453 (2013).
Mast, F.D., Li, J., Virk, M.K., Hughes, S.C., Simmonds, A.J. and Rachubinski, R.A. A Drosophila model for the Zellweger spectrum of peroxisome biogenesis disorders. Dis. Models Mech. 4:659-672 (2011).
Laboratory Members
Research Associate
Dr. Barbara Knoblach
Technician
Hanna Kroliczak