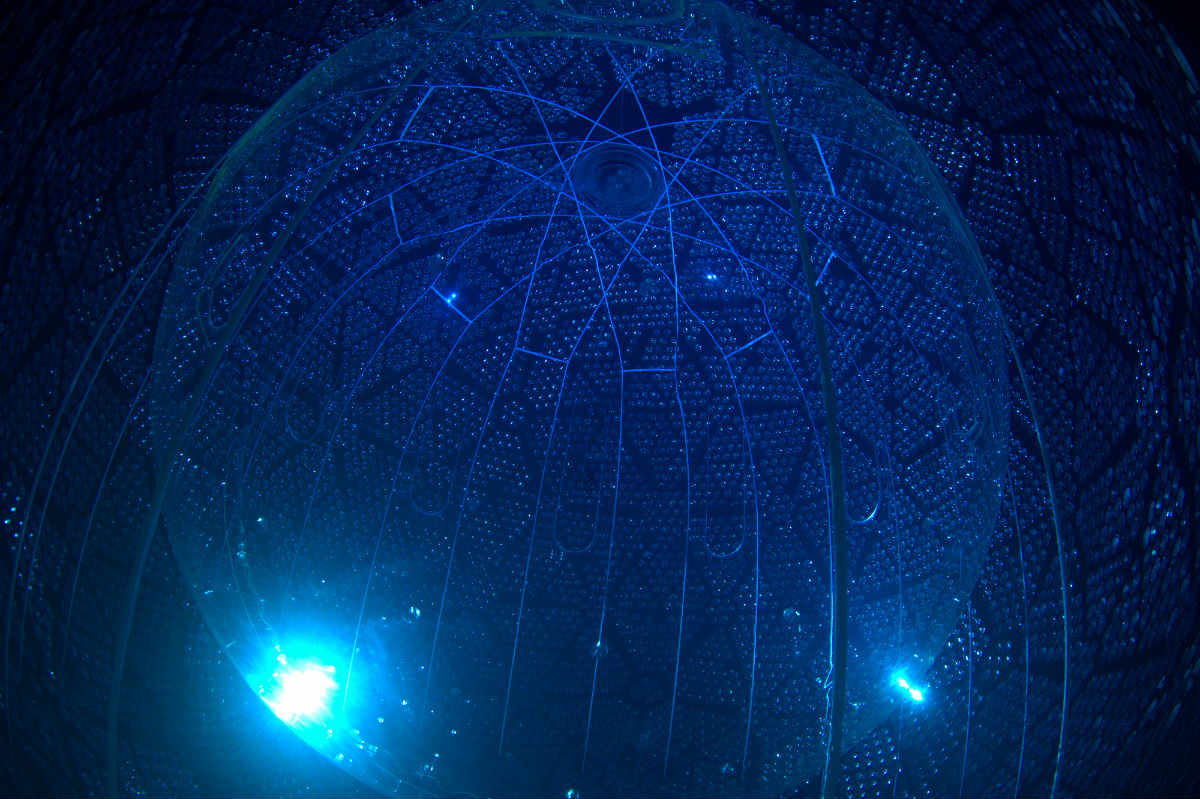
An image from inside the acrylic sphere that houses the SNO+ detector, taken with an underwater camera system developed at the University of Alberta. This precise equipment is being used to detect and measure neutrinos. Photo credit: SNOLAB
Deep underground in Sudbury, Ontario, a sphere floats in a subterranean cavern, ready to detect ghost particles. The equipment at SNOLAB might look and sound like something out of science fiction-but for an international team of scientists, including particle physicists and chemists from the University of Alberta, it's a tool pushing the boundaries of science fact.
After a series of tests requiring incredible precision, the system is ready to push into a new phase of testing that will enable scientists to detect the most difficult-to-detect particles tackled at SNOLAB yet.
The so-called "ghost particles" in question-neutrinos-are closely related to electrons, and the subject of study Carsten Krauss, Juan Pablo Yáñez, and Aksel Hallin, researchers in the Department of Physics.
"We're trying to work out what makes neutrinos tick. They have mass, but we don't know how that mass works," said Krauss, who is also director of the UAlberta Centre for Particle Physics. "SNO+ is designed to figure out if neutrino masses can be measured directly."
The SNO+ detector is located deep underground, housing precise instruments shielded from background radiation-a design that helps reduce interference so scientists can pick up neutrinos.
"SNO+ consists of an acrylic sphere, floating in a cavern that is filled with water," explained Hallin, Canada Research Chair for Astroparticle Physics. "At UAlberta, we were in charge of finding a way to hold the vessel down, which required detailed engineering to create a rope net of ultra-pure material. We also developed an underwater system to monitor the vessel and worked on devices to calibrate it."
When hunting for neutrinos, the composition of materials and finely-tuned calibrations are of critical importance. The sphere itself can be filled with different materials, allowing the team to detect neutrinos of different energy levels.
"The sphere currently contains 900,000 kilograms of water. The next step is to fill it with a material called liquid scintillator-a chemical that converts energy from radiation into flashes of light," said Hallin.
"The primary challenge we face is preparing a suitable scintillator that will be stable for the duration of the experiment. This involves numerous considerations including compatibility with the underground SNO+ facility, understanding the chemistry of the material, preparing candidate scintillators on a lab scale, testing material stability and response, and ultimately implementation in the detector," said Jonathan Veinot, professor in the Department of Chemistry collaborator on the project along with postdoctoral researcher Munish Sharma. "This is a very large undertaking with the potential for huge returns."
With water filling the chamber, the team was able to replicate measurements from a previous series of tests. Those original measurements proved that neutrinos have mass and were recognized with the 2015 Nobel Prize in physics.The new results let the scientists verify that SNO+ is ready for the next phase: using the scintillator to hunt for even more elusive lower-energy neutrinos.
Expect the unexpected
As with any good mystery, the team has also found unexpected results. New theories of particle physics predict a phenomenon called "nucleon decay," where nucleons-protons or neutrons-decay into a lighter subatomic particle.
The SNO+ experiments conducted the most sensitive search for certain types of nucleon decay yet, but were unable able to detect it-proving that if the phenomenon exists, it is even more rare than originally thought and establishing limits on just how infrequent the phenomenon is.
But when pushing the boundaries of particle physics, even determining when a theory needs to be revised is an exciting result.
"There aren't many things in the world of particles that we can't yet properly describe. The standard model of particle physics is very predictive and extremely successful. The masses of neutrinos are not described within this framework," said Krauss. "That's what makes neutrinos so exciting: we learn something new every time we look at a new aspect of how they work."
The papers, "Measurement of the 8B solar neutrino flux in SNO+ with very low backgrounds" and "Search for invisible modes of nucleon decay in water with the SNO+ detector," were published in Physical Review D (DOI: 10.1103/PhysRevD.99.012012 and 10.1103/PhysRevD.99.032008).